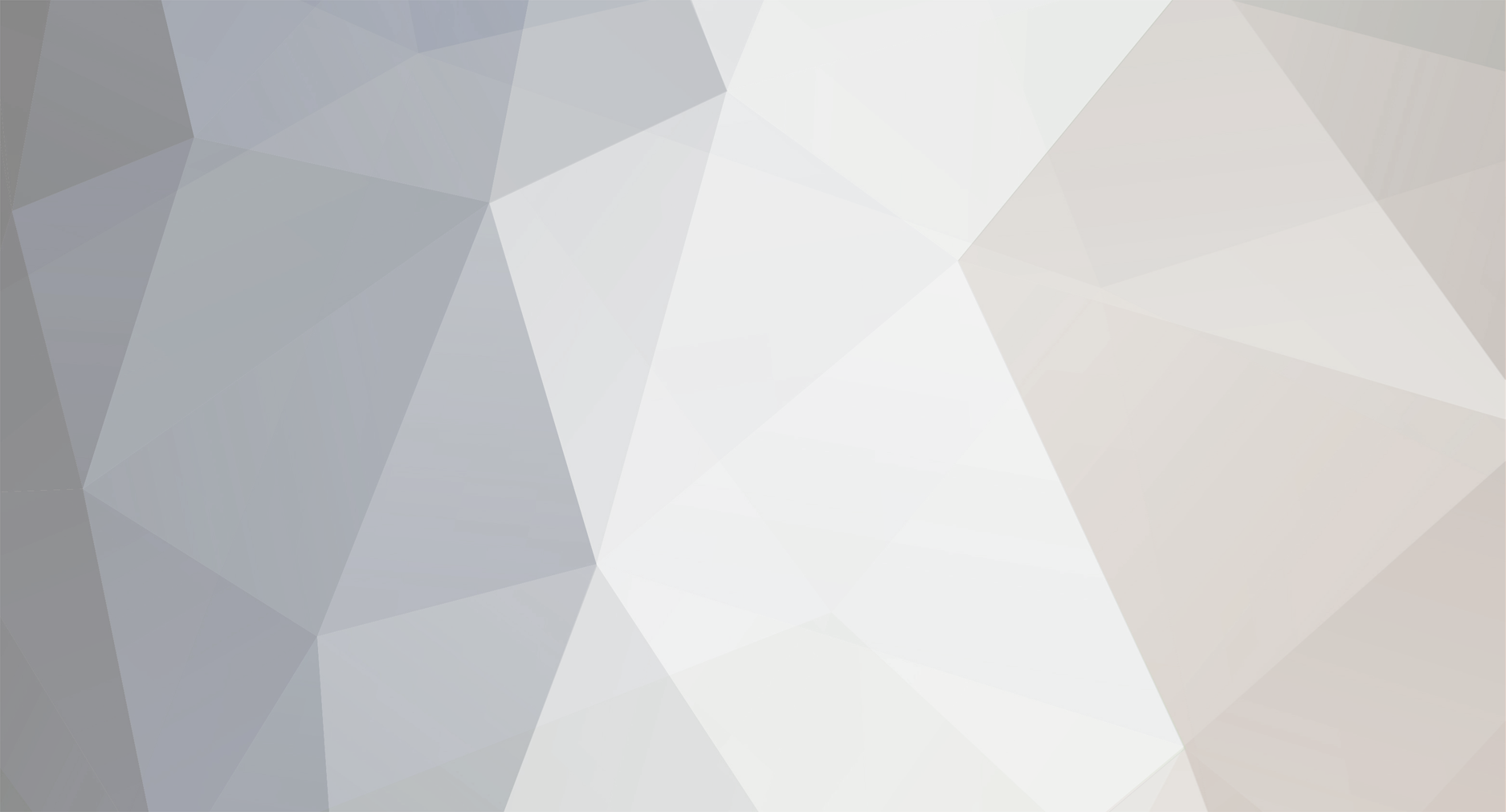
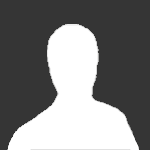
sicivicdude
Members-
Posts
60 -
Joined
-
Last visited
Content Type
Profiles
Forums
Events
Gallery
Store
Everything posted by sicivicdude
-
That particular number was a simple copy and paste from an air cooled motor. You can't push the charge nearly as fast with a higher head temperature. The same basic rules apply for liquid cooled singles, twins, triples etc etc, just that the number is smaller (thus the charge is more energetic) This thread is really different than head design parameters. This is really dealing with just the squish area as that seems to throw some folks for more of a loop.
- 41 replies
-
Head parameters and how they affect power output
sicivicdude replied to sicivicdude's topic in Technical Info
It is easy to tear down someone else, not so easy to express it yourself and I can see how forthcoming you are with your ....er..... vast technical knowledge. You simply tore down what I tried to make relatively simple and easy (IER!) to understand for folks who have no understanding about how it works and offered NO information at all about the subject matter. I defined a term incorrectly and was "taken to task" for it. I don't have a problem at all with someone adding to or even correcting the information available so that everyone has a better understanding (including myself) of the "how" and "why" of it but to simply tear it apart because of one ill defined term is quite narrow minded. While the Webster definition is obviously not as specific as what engine builders will deal with, it nonetheless highlights how undefined the terms are across the board. I didn't aid in this ambiguity any by defining the term incorrectly myself but you can see how easy it is to make the mistake. -
Has anyone seen this abomination?!
sicivicdude replied to MarineNYC's topic in General Banshee Discussion
Not exactly my cup of tea appearance wise but he gets straight 10's across for the board for inventiveness and fab skill, from me! -
The original setup was done as a favor to surfrjag. He happened to run up on a deal with Willard to trade and took it. DDQ's head is next on the "chopping block".
-
"So let's walk through a combustion cycle in a MSV designed head..... It all starts when the transfer ports close (piston coming up)" From the very first post of this thread excluding all of the events that have taken place up to then to get us to the start of the actual compression for the point of explaining how varying squish or quench parameters will affect the combustion process. None the less, I'll indulge. The crankcase on a either reed valved or rotary disced two stroke engine DOES act as the intial "air pump" to get the process started. The reeds also act as the one way check valve in the system to keep it from back flushing. I am agreeing with you there. However, your "simple" explanation that the crankcase does all of the "work" is as flawed as my "simple" explanation of the operation of squish while excluding the operation of the rest of the engine. The point was to explain is as simple terms as possible how changing squish (without changing combustion chamber volume, crankcase volume, port timing, port size, carburetor size, or reed choices) can "tune" how an engine responds to those changes. Internal combustion engines operate as gigantic air pumps. Whether they be 4 stroke engines that open a poppet valve and increase internal displacement (piston falling on the intake stroke), schneurle ported 2 strokes that operate by using the crankcase as their initial air pump, crossflow 2 strokes that operate without the aid of a true tuned pipe (some would argue that the "loop scavenging" of the crossflows acts as a tune pipe but they're not truly balanced so we'll exclude those), Screaming "jimmies" detroit diesel supercharged 2 strokes, or even opposed piston 2 strokes (look up Junkers Jumo 204 or Napier Deltic engines) they ALL operate on pressure differential. Ambient air pressure at sea level is 14.7psi, 1 bar, 760 mmHg nominally. We'll exclude slighty pressure changes due to weather events (although if you want to get REALLLLLLY technical, you should consider rejetting for storms) and call it "nominal". No matter the induction style, pressure differential is how they induct air by moving the air and fuel mixture (except in the case of the diesel!) into the combustion chamber, compressing it, using the force of the explosion to drive the piston down, and exhausting the old mixture. The old "suck, squish, bang, blow" is the really simple version of how it's done. All engine operations are fighting this initial starting pressure (whether they are ultimately forced induction or not) from the moment the air enters the low pressure area around the inlet to the engine (whether that the air filter, airbox inlet, air horn, carburetor horn, turbo inlet, whatever) everything that happens from that point on is a function of restriction versus actual flow. Volumetric efficiency is that measure. That's what I was talking about when I mentioned 14.7 psi as being the starting point. The ability of the engine to actually fill the volume of the combustion chamber to the swept volume of the engine is the "hard fight" on a naturally aspirated engine. Tuned pipes can overstuff the cylinder at their peak operating range but at all others, they don't help. When you leakdown test your engine, how much pressure do you put in there? 7psi. If you apply a lot more than that, you will blow those crank seals out. When the piston is at BDC, both the exhaust port and transfer ports are open. If the exhaust actually operated at 20-50 psi, you would blow crank seals out every time you got on the pipe. I will agree with you that the pipe operates at greatly elevated pressure HOWEVER, only for a brief section and even then only in certain parts of the pipe. During blowdown, the pressure wave from the cylinder passes through the headpipe and divergent section. While that pressure wave is passing, there is a greatly elevated pressure (I doubt 50 psi but definitely higher than ambient) however once the pressure wave enters the divergent section, the relative pressure (pipe pressure to combustion chamber pressure) swings violently back to vaccum! While the transfer ports are open, the pipe begins to actually "draw" charge through the engine directly which is why boyesen ports and the boost port, properly designed, work as well as they do. This is why I say, your "simple" explanation that the crankcase does all of the work is as flawed as mine about squish parameters without including the rest of the operation. That process can actually continue to draw charge directly through all the way until we get back to the start of this thread. That's why I started this thread at that point instead of "muddying" the conversation with what you are discussing. Now, back to the original purpose, do you have any specific information to add about squish or quench areas?
- 41 replies
-
You cannot even begin to compare a 2 stroke "Jimmy" to a schneurle ported 2 stroke gasoline engine. The supercharger HAS to be there or the engine will not operate (not, will not operate properly, just flat out will not run). In a TRUE schneurle ported engine (weedeater for instance) the process you are describing DOES take place (the charge is slightly pressurized by the falling piston) HOWEVER this process does not explain how the engine continues to operate with a boost port or boyesen ports (direct pathways backwards into the intake tract) or how it doesn't pass the entire charge out of the engine while the exhaust port is open. "Higher exhaust pressure"? Do you mean the exhaust wave pulse returning from the tuned pipe? Because again, a scheurle ported 2 stroke engine will still operate without a tuned pipe (chainsaws and weedeaters are fine examples of this) just not to the same tune level as an engine with a tuned pipe. The incorrect term was explained well enough. Pre-ignition or detonation. einstine (Einstein actually) did say that, however, there is very little "simple" about what is going on inside a combustion chamber, even a "simple" 2 stroke engine. The entire point of the thread was to give everyone who don't know any better a little "primer" on how certain parameters effect power output. For someone who knows NOTHING, this is a relatively simple (uh oh, there we go with simple again) method to understand something relatively complicated. Just so there is no confusion, the moderator you all are getting your "information" from is Awk08, the single largest cheerleader for Ken O'Connor Racing out there. The "nut hugging", is 95% him and he does have a chip on his shoulder against me because of some off hand comment made to a fellow KOR groupie.
- 41 replies
-
Check out 2 stroke engine designs before the 1961 grandprix when Suzuki got their hands on tuned pipe technology. They still ran.... Granted, not with nearly as much power output but they run just the same. In fact, if you took your banshee and put a straight pipe on it (no stuffing at all) it would still run (like shit, but run just the same). If you'll read back through, this is for a general understanding of how the engine works and not specific information. Go ahead, tear down the post because I used an incorrect term and not add anything at all about your understanding of how they work. Please, enlighten us as to how they work. I'm sure there are many members who would appreciate you straightening it all out.
- 41 replies
-
I know perfectly well how a 2 stroke works. The entire point of the thread is for people who really don't know any better to be able to read through and get an idea of what the principle is behind the squish area. The entire point of every spark ignited engine is to match the flame front to the piston crown BASICALLY at TDC and push the piston down as the explosion spreads and the pressure rises dramatically. Any preignition on a gasoline engine is detrimental to the power output and engine longevity and should be avoided. I'm not even going to get into diesels deep other than to say that while it may have been the case years ago, when mechanically injected engines ruled the road, that you never had preignition events, each power stroke now can actually have multiple "ignition events" including some well before TDC to "warm up" the compressed air. Those would actually be classified as preignition because the fuel is injected while the piston is still rising and not in order to raise the pressure of the charge in order to push the piston down. Your statement about NEVER wanting preignition ever is absolutely not true. Top fuel engines (I know, we're REALLY stretching the entire point of this thread with this example but I'm making a point that there are very few "laws" of engine building) inject enough fuel to lower the AFR to about 1.5:1. The majority of the fuel entering the cylinder does NOT atomize and enters as a pure liquid. They run ~50 degrees BTDC of ignition timing in order to detonate (and I use that term literally and correctly) the nitromethane that does enter the combustion chamber as an atomized gas. The pressure created by this initial explosion is actually what sets the main explosion off. Liquid nitromethane is an a monopropellant and an explosive under the right conditions. Without detonation, a top fuel engine would not be able to put out the power it does. 14.7 psi is the absolute pressure of air at sea level. When the engine is pulling in air, that's how much it can "grab" by dropping the pressure inside the cylinder to a relative vacuum. When you start speaking to volumetric efficiency of an engine, atmospheric pressure plays a HUGE role in that figure. When discussing the amount of squish a given engine can run, the calculation for volumetric efficiency plays a crucial part in figuring out how much volume is inside the chamber when trying to figure out how fast you can "push" the charge with the piston. Ever heard that as altitude rises compression drops? Ever wonder why? It's not because the physical size of the engine changed....
- 41 replies
-
Head parameters and how they affect power output
sicivicdude replied to sicivicdude's topic in Technical Info
It just means that an spark ignited engine is supposed to be "fired" by the spark plug. Other things can start the ignition event other than the ignition system. Basically, all other sources of ignition (also know as preignition @ sprinklerman) are negative and cause a loss of power or engine longevity. -
Head parameters and how they affect power output
sicivicdude replied to sicivicdude's topic in Technical Info
Agreed, I doubt "Webster" was a gearhead.... Let's just agree that all references to "predetonation" listed apply to all forms of negative parasitic ignition events that are not initiated by the ignition system. -
Head parameters and how they affect power output
sicivicdude replied to sicivicdude's topic in Technical Info
I see what you are referring to but take a look at the Merriam Webster (arguably the final point in all definition arguments) definition: Detonation: 1 : the action or process of detonating 2 : rapid combustion in an internal combustion engine that results in knocking According to their definition, we are both incorrect and the detonation is actually the event you refer to as "pre-ignition" and I am referring to as "predetonation"..... How about we chock that up to a grey area as far as the terms are concerned? Is it safe to say that we can assume that all references in the information listed above to "predetonation" are referring to "detonation" as in the harmful "preignition" of the fuel and air mixture before the normal timed ignition event by the spark plug? -
Head parameters and how they affect power output
sicivicdude replied to sicivicdude's topic in Technical Info
Clearly, that "article" was written regarding blaster heads (where I cracked this nut to begin with) which are fundamentally flawed from the factory. The banshee head is a MUCH better design as far as combustion chamber size, shape, break area, and squish area are concerned. I will be doing a full write up on a stock banshee head maybe tonight so I can tailor the article more directly towards banshee engines. -
Head parameters and how they affect power output
sicivicdude replied to sicivicdude's topic in Technical Info
I'm assuming that you are referring to my seemingly incorrect grammar. If not, please elaborate on your concern. It can also be pre-detonation as the "pre" is a prefix (note prefix DOESN'T use the hyphen), however it is not incorrect to use the term as "predetonation" either. You MUST use the hyphen before anything starting with an "e" but it NOT required before a consonant. Let's not get bogged down in grammar class, however. The point is, there is A LOT going on inside of a combustion chamber while an engine is running. Really, the two threads I've posted are just a "primer" to the real processes anyway in an effort to avoid confusion. -
Head parameters and how they affect power output
sicivicdude replied to sicivicdude's topic in Technical Info
Provided for all those who feel the need to learn WHY and not just be told how..... -
Head parameters and how they affect power output
sicivicdude replied to sicivicdude's topic in Technical Info
Another thing I feel I need to elaborate on is the "overstuffing" of the engine. Volumetric efficiency suffers as engine rpm rises. The same air and fuel has less and less time to make the journey into the engine as the engine turns faster. To this end, anything that can be done to augment the amount or speed of the scavenging can be used to greatly augment the power output at rising engine rpm. We know them as pipes but what they really are is an exhaust driven supercharger. Some are more efficient than others and some have different engine rpm's that they operate at better than others. Generally, pipes operate to "help" the engine not lose power as the time interval for scavenging decreases. However, some pipes actually operate SO efficiently that they can actually put more volume into the combustion chamber at high engine rpm than the engine naturally traps at lower rpm (without the help of the pipe). Drag pipes are a fine example. If one simply calculates the theoretical volumtric efficiency of a drag engine at a given rpm, the output would be dismal. The charge wouldn't have enough time (at the high rpm's) to get the old charge out and new charge completely in before the engine "closed up" and the next combustion cycle began. The tuned pipe is actually so efficient at its job that it can suck the old charge out and even some of the new charge out too and then, due to its design, push that new charge back in before the exhaust port closes. If the pipe is good enough at its job that it does manage to get more charge into the cylinder than can be trapped at lower rpm's, it is overstuffing the engine. It's not uncommon to see highly tuned race engines exceed 110% of their "theoretical" volume. A 100cc combustion chamber (the area above the exhaust port) could potentially contain 110 cc's of fresh charge before the piston compresses it into the combustion chamber and even begins the squish phase of the stroke... This is another reason why head designs for "most" engines are kept "safe" instead of to the raggedy edge of their possible design. Change elevation with the exact same engine (no chagnes at all) and even rejetting may not be able to help predetonation that could come on from the increased volumetric efficiency. -
Head parameters and how they affect power output
sicivicdude replied to sicivicdude's topic in Technical Info
I said that not all predetonation is audible. What I mean by that is that the rider would not necessarily be able to hear "knock" at low levels and by the time the rider can hear and diagnose the "knock" the engine has been predetonating for quite some time. Possibly to the detriment of the engine. An improperly designed head can predetonate and hammer away at the big end and small end bearing forever and barely be audible outside of the engine. Doesn't mean it can't damage the rotating components. Install a knock sensor on the side of the motor and THEN test for predetonation if one should choose to "experiment" their way to hard squish numbers. However, I caution against that too, as soon as you have hard squish data numbers and change one parameter you are no longer perfectly optimized again. The lesson to take away from this post is that specific numbers are only specific for a specific build. More general numbers are slightly less powerful (still significantly more so than factory heads) but also "safer" for more conditions and mishaps. Also, it's easier to discuss and understand if we all get on the same page about the process of design and building of a rechambered head. It was suggested I include some more information (all of which is true and relavent!) Number one is that the faster, more energetic charge burns faster. This has the same basic (flame hits the piston earlier) effect as advanced ignition timing. Igniting a lazy charge early and igniting a really excited charge later end up at the same point. Generally, there is less (or even no need at all) need to run advanced ignition timing with a rechambered head. Second thing is that the value (increased charge speed) diminishes somewhat with increased compression. At some theoretical point, the compression will be so high, that the squish area isn't "exciting" the charge any more than it would be naturally using the higher compression. This limit, I don't believe, is applicable to air cooled singles as heat and fuel choice is the limiting factor on these engines anyway, but an interesting note none the less. Some er...... light reading on the subjects (and many many more). SOME of the data, theories, and methods used are slightly outdated (engine's designed in the 60's and built in the 70's) but generally it still applies in theory and concept! A reference of books: Design and Simulation of 2 Stroke Engines, Gorden P Blair 1996 Basic design of the Two Stroke Engine, Gorden P Blair 1990 Two-Stroke Tuner's Handbook, Gordon Jennings, 1973 Two Stroke Performance Tuning, Alexander Graham Bell, 1999 The High Performance Two Stroke Engine, John Dixon, 2005 -
Head parameters and how they affect power output
sicivicdude replied to sicivicdude's topic in Technical Info
Third thing we have to consider when designing a optimized head is the squish band area (differing from the "squish area", the name for that area). Here, meaning the squish percentage. This is very important when calculating the squish gap or "thickness" because as you push a given amount of charge (as estimated by the CCR) at a certain RPM (as astimated by the pipe, porting, and carb setup) the distance that the charge must travel to reach the center of the combustion chamber imparts more energy on that charge. You can have a 40% by area head that can safely run a .040" but a 50% by area head needs more gap to keep the same squish velocity. Remember, velocity = energy. The more energetic the squish area is, the better, more vigorous, and stronger the combustion fire is going be. However, if it charge explodes before the piston comes all of the way up to the top, you're wasting that compression and potentially doing a lot of damage to the reciprocating mass of the engine! High rev engines (drag/ dune motors) need less percentage. Simply put, a smaller squish percentage means better scavenging of the last charge (less burnt up fuel and air left in the head) but it also means a slightly less energetic "push" from the squish area. Lower rev engines can get a great torque advantage by having a larger squish percentage because the slower piston speed means not TOO much velocity in the charge. Most engines (gasoline trail bikes for example) do well with 50% squish percentage. In other words, the squish band is 50% (by area, NOT diameter!) of the bore area. There are a few more things to consider: The size of the "break area" makes a lot of difference to predetonation resistance, actually. The aluminum in the break area is at the junction of all of the energy being put into the charge by the rising piston and all of the heat being generated by the explosion. Considering 2 strokes never get a "dead stroke" to cool off, too sharp a break area means some aluminum is "sticking out" into the combustion chamber with less material around it to "wick" that heat away. A super sharp break area can be quite a bit hotter than the average temperature of either the squish area or combustion chamber! A less rounded break area is good for keeping the squish velocity the highest for the longest period of time HOWEVER they're prone to predetonation. Really wide break areas, are less prone to predetonation but also allow the squish velocity (and the energy imparted into the charge) to diminish as the charge passes out into the combustion chamber. -
Head parameters and how they affect power output
sicivicdude replied to sicivicdude's topic in Technical Info
Second thing that affects the head design is exhaust port duration. This number GREATLY affects the Corrected Compression Ratio. Only stating head volume without knowing the exhaust port duration or CCR (either will work) is like knowing the bore and stroke of one cylinder without knowing how many cylinders there are! The exhaust port duration is used to calculate the CCR which will tell us how much charge is actually getting pushing into the trapped volume. This is very important to know because it will affect the final cranking compression numbers. A higher amount of exhaust port duration means that less charge is trapped inside the combustion chamber by the rising piston. Less charge trapped means that the final CCR is lower. While this *GENERALLY* (we'll get back to this later!) means a lower CCR and less engine performance, there are obvious benefits. Longer exhaust port duration means better scavenging of the last charge (the fresh charge from the carburetor "pushes" the last charge out the exhaust port). The total trapped volume needs to be considered with regards to exhaust port duration. The head volume can compensate for the lower CCR by lowering the trapped volume to keep the CCR constant (or increase it). -
Head parameters and how they affect power output
sicivicdude replied to sicivicdude's topic in Technical Info
So this leaves us with a conundrum. In order to correct the deficiencies in the stock head design, we must correct the squish band for angle, remove some of the mating surface, and remove some of the combustion chamber material (to correct the volume). This is how a stock head, ends up looking like this: Now, in order to make the changes properly, one must know several things. First off, the intended use of the engine (and quad really) comes into play. The reason is this; the maximum squish velocity of a given head with a preset squish gap is effected the greatest by RPM. If you completely optimize a head shape and install a higher rev pipe that will allow another 1k rpm of over-rev you WILL get predetonation in that head. It may not be enough to be audible but it will be there. NOT ALL PREDETONATION IS AUDIBLE. BY THE TIME YOU CAN HEAR IT, IT HAS ALREADY BEEN HAMMERING THE ROTATING MASS FOR A LONG TIME! Tuning a head by "listening" for predetonation is also foolhardy and will end in disaster over the long haul. -
Head parameters and how they affect power output
sicivicdude replied to sicivicdude's topic in Technical Info
Alright so the first thing we need to understand is that head design is very subjective. It's extremely difficult to compare squish, combustion chamber volume, or really any of the other parameters without knowing all of the parameters and how the affect each other. In this case knowledge is power (QUITE literally). First off, lets dispell a myth. An "smaller" cc head is NOT ALWAYS BETTER than a "larger" cc head. That method of "comparing" head sizes is fundamentally flawed. An 18cc head on a stock exhaust port engine will knock like hell while a 20cc head on a drag ported cylinder running methanol will barely be adequate to fire off the fuel. Comparing ONLY volume is a dangerous and foolhardy method of comparing head sizes and shapes simply because so many other things compound head shape other than volume. Also, "head volume" is NOT the same thing as trapped volume. Trapped volume is actually more important than head volume (which is only semi useful in comparing head to head to head, not engine build to engine build!) because that is what is used to calculate the UCCR or CCR. The piston is domed and properly built, the trapped volume will actually be less than the head volume! We, being relatively intelligent creatures will move past comparing "dome size" as a comparison for engine tune level (or even head tune level). The single biggest problem with the stock blaster head is that the squish band is cast at a different angle than a stock piston. A stock stroke stock bore engine (we'll discuss that before we get into strokers and big bores!) has a negative deck height of approximately -.008". Head gasket thickness is a nominal .032". The stock head has a positive step (further away from the piston) in it of something like .055" and an angle of approximately 20°. The piston has an angle of approximately 10° (averaged over the outside 50% of the bore size). To begin with, the stock engine closest squish point of approximately .095" or 2.4mm. From there it actually growsin thickness up to approximately 4mm before hitting the break area. JUST shaving off the step until the mating surface is flush with the outside edge of the squish band will yield a relatively close .040" squish at the very outside edge growing larger as it goes. This will, at the very outside edge, push the charge very energetically but with diminishing force as it goes. The very energetic push at the start can actually cause an otherwise completely stock engine to predetonate. The first step to all head mods is to recut the squish angle to ~11°. This process alone, however would yield a squish gap of something like .200" (roughly 4 times the amount desired) and a combustion chamber volume of something like 24 cc's but only because the squish would be sooooo large. That head would run worse than a stock head because it's hardly pushing the charge towards the spark plug at all. Removing the sealing surface to correct the squish gap would yield a head volume of something really tiny like 16cc's which would run great, for about 4 minutes until the engine heat soaked. At which point the glow plug (spark plug but with enormous amounts of charge heat) would begin to predetonate the charge and eventually tear up the engine. This might be optimal for a quad that will never get hot enough to knock? Maybe? Not very useful for most folks.... -
First, this is in addition to the information included in "squish, mystery or not" thread. You'll need to cover some of that ground first to understand how squish in particular affects the other parameters and why the other parameters change the needed squish. Glossary: Squish or quench area or band: The area between the outside edge of the combustion chamber and the hemispherical (or torrodial) area in the center of the head. Squish gap/clearance: The distance between the squish area and the piston crown at TDC. Combustion chamber: The area inside the head that the flame and fire contact. Usually consists of two general areas, the squish or quench area and the chamber proper. Squish percentage: The percentage of the total bore area that is taken up by the squish band and "break" area. Break area: The small amount of material that is used as a transition area from the squish area to the combustion chamber. UCCR (UnCorrected Compression Ratio): The mathematical calculation representing the ratio of swept volume to trapped volume in an engine. Swept volume: The volume that the moving piston displaces as it travels. Basically, bore x stroke = displacement. Trapped volume: The area that all of the swept volume is compressed into when the piston reaches TDC CCR (Corrected Compression Ratio): The mathematical representation of volume above the exhaust port to trapped volume. Usually called the "Japanese method" and only useful for cranking compression comparison on 2 stroke motors! TDC: Top Dead Center the upmost point that the piston travels, when the crank bearings, crank pin, big end bearing, piston pin bearing, and piston thrust are all aligned "straight up and down". Crucial figure for head tuning as it's needed to figure out squish gap thickness BDC: Bottom Dead Center. The lowest point that the piston travels to. Predetonation: The term that refers to when the charge is fired too early. It takes a certain amount of time for the explosion (or more precisely, the pressure wave generated during the explosion) to reach the piston crown. This is the reason the stock ignition timing is "advanced" during higher RPM operation. The time it takes that flame front and pressure wave to hit the piston is constant (relatively) but the piston speed and dwell time is more and more limited as rpm's rise. Predetonation can occur due to either too much ignition timing (controlled predetonation that can be adjusted out) or by other factors present in the engine (too huch heat, lean condition, "hot spots") Dwell: In modern engines, the amount of time and position of the piston while the connecting rod is rolling over and under TDC and BDC. The piston also changes face thrust at this position. In a well worn cylinder, there is a "groove" at the top and bottom of the cylinders where the rings rub the cylinder at the dwell points. This is the area with the most amount of wear in a "normal" engine. Squish velocity: The speed of the homogenized charge (air:fuel mixture) as the piston rises and "pushes" the charge towards the center of the combustion chamber. Pushing the charge too fast (high velocity) will preheat the charge to the point of autoignition resulting in predetonation. Piston speed affects this number GREATLY. Piston speed: The rate at which a piston rises and falls for a given crankshaft RPM. Usually expressed as feet/second. More rpm = greatly increased piston speed. Overstuffing: The rate at which volumetric efficiency rises well beyond "normal" capacity as the engine, pipe, carb, portwork, and head begin to work together. Volumetric Efficiency: The ratio of the engine's ability to move old charge out and new charge in under real world conditions versus the "theoretical" ability at no engine rpm. Generally given as a percentage that gradually drops as engine rpm increases.
-
Yes Matt, I did ask if it was OK to repost some of my learned knowledge over here too and got the go ahead. I didn't feel like posting a linkback so I copied my parts of the thread and posted them over here. Feel free to add your own information to the thread.
- 41 replies
-
There are three methods to measure the squish area. One is the "recommended" way but it has a drawback... You can cut two pieces of thick plumbing solder and insert them into the spark plug hole with the engine assembled and rotate the engine around so that the piston squishes the solder flat and then remove the solder and measure it. The disadvantage is that the solder that is large enough diameter to be used successfully for squish testing is generally acid cored. Squishing that is going to squirt acid out onto the piston, cylinder, and head. Not a problem if you plan on running it the same day. Kind of an issue if you are going to leave it for a while! The second method is to insert some heavy modellers clay onto the top of the piston, install the head, and then roll the motor over. This squishes the clay flatter around the outside. The disadvantage is that it requires removal of the head after the squishing has taken place to measure the clay thickness around the outside edges and the clay has to be handled VERY carefully in order to measure. The third method is purely mathematical. You measure how far down (or up) the piston is inside the cylinder with a squished head gasket installed. Then you measure the "lip" (or lack there of) of the head and add the two together. The distance between the piston crown at TDC and head is the squish thickness. All three methods have some drawbacks, obviously but they all can work equally well if you pay attention to what you're doing and take your time!
- 41 replies
-
Now, there are several programs out there to handle the calculations (not that this sort of thing is "normal" for a rider to know) but the jist of the situation is this: Engine rpm, squish band "depth", squish band angle, and squish band height (above the piston crown) all go into figuring how "hot" the charge is going to get as you push it outwards. The faster the engine is turning (the upper most point that the pipe/porting/carb will ever allow the engine to run) the faster the piston is coming up and the more pressure it can generate faster. Since temperature rises with pressure, the faster you generate a pressure wave, the hotter the gas will get as the piston pushes it. If you have an area of equally distributed fuel and air, the farther you have to push it, the more you are going to push against and the more pressure is going to be generated by a given squish clearance. There are functions to the efficiency gains (and losses) about making the squish area more of the total bore but not as "tight" or less of the bore but "closer". The gains basically are tied to the ability of the squish area to quench the fire out around the edges of the cylinder at TDC versus pushing the charge too fast. The "magic number" is actually a simple calculation of about 50% of the bore size (in area) being just about right. Obviously, in order for the quench area to do its job, the layer of gas inside the squish area at TDC needs to have the maximum contact area with the "cool" head and piston crown. If you have the squish area at an angle that's too great, the pressure wave created during the squish phase is too weak to excite the charge properly and the squish zone towards the middle of the combustion chamber isn't tight enough to dampen out the flame. (THIS IS THE STOCK YAMAHA BLASTER HEAD DESIGN!) If the squish area is angled too shallow, the pressure wave is actually squished into a narrower area as the piston rises with the pressure skyrocketing and the likelylihood of predetonation going right along with it. The "sweet spot" of squish angle is just a tiny but more than the piston crown. Blaster pistons along the outside 50% of the piston crown have a nominal angle of 10 degrees. Most squish areas are cut to about 11 degrees to avoid any chances of the squish area converging and creating a hot spot. Squish band height is what I've already covered really..... the distance from the piston crown to the cylinder head around the outside 50% of the cylinder. Already covered why too tight and too loose is bad.
- 41 replies
-
We'll start off with too much squish (too much area between the piston and head at TDC). This isn't a particularly dangerous situation and it's how the stock yamahe head is setup. Basically, the only issue is that you are leaving power on the table. If you take a given octane rating gasoline at a perfect stoich mixture rate (14.7 for standard non-oxygenated gasoline) and heat it to a given point JUST below the point that it will spontaneously combust, the resulting explosion when it does go off will carry more energy with it (push the piston harder) and more completely use up all of the fuel (combustion efficiency). The magic point when you are exciting the mixture of hydrocarbon molecules TOO hard is impossible to measure exactly without destroying the sample (burning it ) which is done using a variable compression engine (usually by government agencies charged with making sure that gas stations aren't cheating). What you can do is generalize about fuel quality by using the MON number (octane rating) at the pump and build the engine around that. What we call a "pump gas engine" is built differently (specifically in the head) than a "race gas" engine because race gas is less likely to detonate on its own when it's pushed very fast. Too large a squish area won't push that fuel/air mixture fast enough to heat it to just below the spontaneous combustion temperature and the resulting fireball during the ignition event won't carry as much energy. Too small a squish area has the opposite effect but MUCH worse results.... As the piston is rising and nearing the top of the stroke, the pressure inside the mixture around the outside of the cylinder is spiking. Because pressure and temperature are absolutely tied together, (See: Ideal Gas Law!) the termperature in that area also spikes. Once the temperature in that area spikes to above the auto-ignition temperature, a flame breaks out. Because the fuel in this area is superheated and unstable, a small torus (think, doughnut) of high pressure high temperature fuel/air mixture begins to overpressure the area inside the squish area. The rise in pressure is, unfortunately, confined rather well by the squish area and pushes outwards onto the cylinder walls, head, and down onto the piston. The last is the MOST devastating as the piston is still moving upwards towards the heat and pressure, all of that force is directed into the crown (believe it or not, slight plastic deforming the piston crown!) of the piston, wrist pin bearing, wrist pin, down the rod, big end bearing, crankshaft, main bearings, and finally into the cases. Enough "knocking" will eventually chew all of the bearings up or worse, ruin the main bearing seating areas and that set of cases forever (suzuki LT's are known for this!)
- 41 replies